Creating Electricity with Caged Atoms
At the Vienna University of Technology, a new class of thermoelectric materials has been discovered. Due to a surprising physical effect they can be used to create electricity more efficiently.
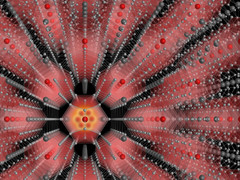
Clathrates: crytals enclosing single atoms
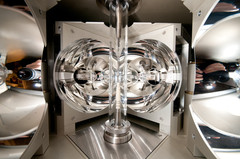
The mirror oven at TU Vienna, in which the novel material was created
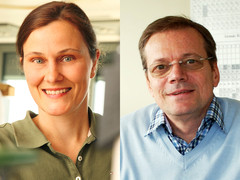
Prof. Silke Bühler-Paschen and Prof. Andrey Prokofiev
A lot of energy is wasted when machines turn hot, unnecessarily
heating up their environment. Some of this thermal energy could be
harvested using thermoelectric materials; they create electric current
when they are used to bridge hot and cold objects. At the Vienna
University of Technology (TU Vienna), a new and considerably more
efficient class of thermoelectric materials can now be produced. It is
the material’s very special crystal structure that does the trick, in
connection with an astonishing new physical effect; in countless tiny
cages within the crystal, cerium atoms are enclosed. These trapped
magnetic atoms are constantly rattling the bars of their cage, and this
rattling seems to be responsible for the material’s exceptionally
favourable properties.
Cerium Cages from the Mirror Oven
“Clathrates”
is the technical term for crystals, in which host atoms are enclosed in
cage-like spaces. “These clathrates show remarkable thermal
properties”, says Professor Silke Bühler-Paschen (TU Vienna). The exact
behaviour of the material depends on the interaction between the trapped
atoms and the cage surrounding them. “We came up with the idea to trap
cerium atoms, because their magnetic properties promised particularly
interesting kinds of interaction”, explains Bühler-Paschen.
For a
long time, this task seemed impossible. All earlier attempts to
incorporate magnetic atoms such as the rare-earth metal cerium into the
clathrate structures failed. With the help of a sophisticated crystal
growth technique in a mirror oven, Professor Andrey Prokofiev (TU
Vienna) has now succeeded in creating clathrates made of barium, silicon
and gold, encapsulating single cerium atoms.
Electricity from Temperature Differences
The
thermoelectric properties of the novel material have been tested.
Thermoelectrics work when they connect something hot with something
cold: “The thermal motion of the electrons in the material depends on
the temperature”, explains Bühler-Paschen. “On the hot side, there is
more thermal motion than on the cold side, so the electrons diffuse
towards the colder region. Therefore, a voltage is created between the
two sides of the thermoelectric material.”
Experiments show that
the cerium atoms increase the material’s thermopower by 50%, so a much
higher voltage can be obtained. Furthermore, the thermal conductivity of
clathrates is very low. This is also important, because otherwise the
temperatures on either side would equilibrate, and no voltage would
remain.
The World’s Hottest Kondo Effect
“The
reason for these remarkably good material properties seem to lie in a
special kind of electron-electron correlation – the so-called Kondo
effect”, Silke Bühler-Paschen believes. The electrons of the cerium atom
are quantum mechanically linked to the atoms of the crystal. Actually,
the Kondo effect is known from low temperature physics, close to
absolute zero temperature. But surprisingly, these quantum mechanical
correlations also play an important role in the novel clathrate
materials, even at a temperature of hundreds of degrees Celcius.
“The
rattling of the trapped cerium atoms becomes stronger as the
temperature increases”, says Bühler-Paschen. “This rattling stabilizes
the Kondo effect at high temperatures. We are observing the world’s
hottest Kondo effect.”
More Research for Better and Cheaper Clathrates
The
research team at TU Vienna will now try to achieve this effect also
with different kinds of clathrates. In order to make the material
commercially more attractive, the expensive gold could possibly be
substituted by other metals, such as copper. Instead of cerium, a
cheaper mixture of several rare-earth elements could be used. There are
high hopes that such designer clathrates can be technologically applied
in the future, to turn industrial waste heat into valuable electrical
energy.
Original Publication
Picture Download
Further Information:
Prof. Silke Bühler-Paschen
Institute of Solid State Physics
Vienna University of Technology
Wiedner Hauptstraße 8-10, 1040 Vienna
T: +43-1-58801-13716
silke.buehler-paschen@tuwien.ac.at
Prof. Andrey Prokofiev
Institute of Solid State Physics
Vienna University of Technology
Wiedner Hauptstraße 8-10, 1040 Wien
T: +43-1-58801-13113
andrey.prokofiev@tuwien.ac.at